How Do We Search for Intelligent Life?
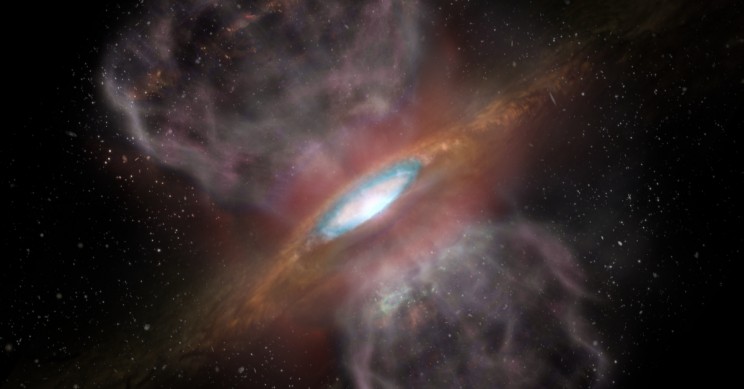
After several decades, the Search for Extra-Terrestrial Intelligence (SETI) continues to look for life beyond our Solar System. Improved instruments and more refined methods are likely to make a big difference. But in the meantime, the question remains: “where is everybody?”
For centuries, humanity has been captivated by the idea of finding intelligent lifebeyond our planet.
In many ways, this is a consequence of astronomers discovering that the planets of our Solar System were not that different from Earth, as well as the fact that our Sun is one of the billions within our galaxy (and our galaxy one of trillion within the cosmos).
However, it has only been within the past century that any efforts to find life beyond Earth have been mounted. These efforts are largely the result of the development of radio astronomy, where radio antennas are used to detect radio waves from cosmic sources.
In the past few decades, our methods have grown and matured, to the point where we are able to search far and wide for signs of life.
But as Enrico Fermi famously said, “where is everybody?” In other words, if the possibility of intelligent life is even remotely possible, why has humanity not found any evidence of life out there?
This question is not only the basis of the famous Fermi Paradox, but it also lies at the heart of humanity’s ongoing Search for Extra-Terrestrial Intelligence (SETI).
So how are we going about this task? What are our methods and what kinds of limitations we are working with? And while we’re at it, just how likely are we to find evidence of extra-terrestrial intelligences (ETIs) in our galaxy or the Universe at large?
The Drake Equation:
In 1961, Cornell astronomer Francis Drake proposed his famous equation for calculating the number of extraterrestrial civilizations in our galaxy that we would be able to communicate with at any given time. Known as the Drake Equation, this formula for determining who could be out there has informed a great deal of humanity’s SETI research efforts.
The equation is expressed mathematically as N = R* x fp x ne x fl x fi x fc x L, where:
N is the number of ETIs that we might be able to communicate with
R* is the average rate of star formation in our galaxy
fp is the number of stars that have a system of planets
ne is the number of planets that will be able to support life
fl is the number of planets that will develop life
fi is the number of planets that will develop sentient (aka. Intelligent) life
fc is the number of civilizations that will develop advanced technologies
L is the length of time that these civilizations will have to transmit radio or other communications signals into space
What is encouraging about the Drake Equation is that, even when it is adjusted for very conservative values, the number of ETIs that would exist in our galaxy is still significant. For example, scientists estimate that there are between 200 and 400 billion stars in our galaxy, with new stars forming all the time. In addition, recent work in the extrasolar planet (aka. exoplanet) research has revealed that most star systems are likely to host at least one planet.
These same research efforts have also found many exoplanets that exist within their stars circumsolar habitable zone (aka. “Goldilocks Zone”), the region where temperatures are warm enough to support the existence of liquid water on the planet’s surface.
These discoveries have indicated that within a fair number of star systems, there are worlds that are capable of supporting life as we know it.
So even if we assume that only 1% of all planets in our galaxy is capable of supporting life, and only 1% of those will develop life, and only 1% of those will develop advanced technology, etc. etc., and that these civilizations will only have a few thousand years before they go extinct, we still come up with results that say that there should be at least a few alien civilizations out there at any given time.
Unfortunately, and as you can probably tell, the equation is subject to a lot of uncertainty. For starters, we have no idea how many “potentially habitable” planets are statistically likely to give rise to life. The same holds true for how many planets will support the development of complex life, let alone sentient life, or how many of those will be able to develop technologies that we would be able to recognize.
When you take all that into account, you begin to understand why SETI is such though, research-intensive business. Basically, scientists are looking needles in the cosmic haystack, but are uncertain whether or not those needles will be recognizable.
So the question remains, how exactly are we looking for signs of extra-terrestrial intelligence in the cosmos? And would we even recognize it if we saw it?

SETI vs. METI:
First, an important distinction needs to be made when it comes to the search for ETIs, and that is the difference between passive and active measures.
Whereas passive measures describe just about all SETI efforts to date, there have also been several attempts to send out messages that would be detectable by ETIs. This is what is known as Messaging Extra-Terrestrial Intelligence, or METI.
Whereas organizations like the Search for Extra-Terrestrial Intelligence (SETI) Institute and many radio observatories around the world have been dedicated to listening for signs of intelligent life for generations, non-profit organizations like Messaging Extraterrestrial Intelligence (METI) International are less numerous and take a more active approach.
And whereas the term SETI seems to have emerged over time, the term METI was coined by Russian scientist Alexander Zaitsev, who sought to draw a distinction between passive and active methods to find evidence of extra-terrestrial civilizations. As Zaitsev explained in a 2006 paper on the subject:
“The science known as SETI deals with searching for messages from aliens. METI science deals with the creation of messages to aliens. Thus, SETI and METI proponents have quite different perspectives. SETI scientists are in a position to address only the local question “does Active SETI make sense?” In other words, would it be reasonable, for SETI success, to transmit with the object of attracting ETI’s attention? In contrast to Active SETI, METI pursues not a local and lucrative impulse, but a more global and unselfish one – to overcome the Great Silence in the Universe, bringing to our extraterrestrial neighbors the long-expected annunciation ‘You are not alone!’”

It is also worth mentioning that while SETI and METI efforts are interrelated and have evolved over time together, the former predates the latter by several decades and it is only in recent years that METI has begun to stand out on its own. In addition, there are unresolved questions regarding the effectiveness and even safety of these methods. For instance, is it even a good idea to let potential ETIs know where we live, assuming that they might not be entirely friendly?
However, those are questions that will have to wait for another day. In the meantime, there’s the more pressing question of how we are looking for ETIs and what we are looking for. These can be broken down into two broad categories, which amount to looking for signs of life (biosignatures) and signs of activity (technosignatures).
Searching for “Biosignatures”:
Because of the rather significant challenges associated with searching for life beyond our Solar System, scientists are forced to take what is known as the “low-hanging fruit approach.” This amounts to looking for signs of “life as we know it,” which are collectively known as biosignatures.
Biosignatures, simply put, are telltale indicators that life could exist on a planet or celestial body. These can include certain types of atmospheric elements (oxygen gas, carbon dioxide, methane, etc.), stable isotopes, minerals, organic molecules, pigments, or anything else that scientists associate with biological processes.
For example, astronomers often try to obtain spectra from the atmospheres of the exoplanets in order to determine their chemical compositions. This is sometimes possible using Transit Photometry, where a planet passes in front of its star relative to the observer. These transits allow astronomers to determine the presence, size, and orbit of an exoplanet.
But sometimes, under the right circumstances, the light passing through the planet’s atmosphere also allows for spectroscopic studies that let astronomer’s know what chemical compounds are there. The presence of oxygen gas in a planet’s atmosphere is not only seen as an indication that it could be “potentially-habitable”, but also inhabited. This is because on Earth, oxygen gas is a byproduct of photosynthesis – a biological process. Much the same is true of carbon dioxide.
Whereas planets and simple bacteria consume carbon dioxide via photosynthesis and produce oxygen, mammalian life forms consume oxygen gas and produce carbon dioxide as a waste product. Methane is also a waste product, the result of certain mammals digestive process (i.e. cows), and is released when organic matter decays.
Scientists also hope to use next-generation telescopes to study more exoplanets using a process known as Direct Imaging. As the name suggests, this is where scientists are able to detect a planet by capturing light reflected from its surface and/or atmosphere, rather than inferring its presence through indirect means – such as Transit Photometry or the gravitational influence exoplanets have on their stars (aka. the Radial Velocity Method, or Doppler Spectroscopy).
If scientists are able to obtain spectra directly from a planet’s surface, they will be able to see what wavelengths of light are being absorbed and which ones are being radiated. This method, which was endorsed by famed astronomer Carl Sagan, could provide evidence of life by indicating the presence of photosynthesis. Thanks to decades of Earth observation, scientists have come to understand that green vegetation can be identified from space using what’s called the Vegetation Red Edge(VRE).
This phenomenon refers to how green plants absorb red and yellow light while reflecting green light, while at the same time glowing brightly at infrared wavelengths. At the same time, retinal-based photosynthesis (which may predate chlorophyll-based photosynthesis) absorbs most strongly in the green-yellow parts of the spectrum and emits purple light. In this way, more basic forms of photosynthesis could be identified by looking for purple light.
Searching for “Technosignatures”:
In the same way that biosignatures are indications of possible life on another planet or celestial body, technosignatures are indications of possible technological activity. In this respect, scientists are on the lookout for technologies that we know work (because we’ve tested them ourselves) or are at least scientifically feasible.
Of all the technosignatures to be considered and investigated, radio communication signals are the most time-honored. However, many other methods have been proposed based on technologies that are known to be feasible (or are at least theoretically so). For example, in September of 2018, the NASA Technosignatures Workshop took place in Houston. By November, the workshop’s report was released, titled “NASA and the Search for Technosignatures.”
According to the report, while no evidence has been found of radio signals that were clearly artificial in origin, radio astronomy remains a viable means of searching for ETIs. In much the same way, directed energy (laser) signals have been considered as a possible means of communication and/or propulsion.
Proponents of this include Professor Philip Lubin of the University of California Santa Barbara (UCSB) and Professor Abraham Loeb of Harvard University. Both professors are proponents of using directed-energy as a means of spacecraft propulsion and are intrinsically involved in the first attempt to create such a spacecraft – Breakthrough Starshot.
The proposal calls for the use of lasers to accelerate a lightsail up to speeds of 20% the speed of light, which would allow it to reach Alpha Centauri in just 20 years. As Lubin explained in a recent research paper, this technology could also be considered a technosignature. Much as Lubin and fellow researchers have been working on directed-energy concepts for the sake of propulsion, planetary defense, scanning, power beaming and communications.
Assuming that ETIs use directed-energy for similar purposes, astronomers could observe nearby star and planetary systems for signs of “spill-over” (i.e. errant flashes of laser energy) or possibly even laser beacons. Other means of communications that have been proposed range from neutrinos to Fast Radio Bursts (FRBs), and even include gravitational waves.
As already noted, megastructures are also considered a viable technosignature. The concept was popularized during the 1960s thanks to the work of scientists like English-American theoretical physicist Freeman Dyson and Russian astrophysicist Nikolai Kardashev. In 1960, Dyson released a paper titled “Search for Artificial Stellar Sources of Infrared Radiation”, which proposed that intelligent species could eventually create megastructures that would be able to harness the energy of their stars – henceforth known as “Dyson Spheres” or “Dyson Structures”.
Other megastructures that have been proposed include Ringworlds (or Niven Ring, after Larry Niven), which consist of one or many rings orbiting a star. There’s also the Matrioshka Brain proposal by science fiction writer Robert Bradbury, who foresaw a massive computer made up of several Dyson structures arranged one inside the other (like a matryoshka doll) that used a star’s energy as a power source.
There’s also the Stellar Engine (aka. Shkadov Thruster), where a megastructure is built around the star in such a way that it is able to focus the star’s solar wind in one direction, thus generating propulsion and moving the star. And then there’s the Alderson Disk (or Disk World), proposed by NASA scientist Dan Alderson, where a massive, flat disk would be built around the star to maximize habitable space.

In 1963, based on work with Russian SETI efforts, Kardashev created a three-tiered classification system for intelligent species based on their level of technological development. Known as the Kardashev Scale, this system ranked species based on its ability to harness the energy of their planet, solar system, and galaxy, respectively. The ability to harness this energy would theoretically rely on megastructures of increasing complexity and enormity.
Efforts to detect such structures have included infrared astronomy (as Dyson recommended), which would consist of identifying megastructures around stars based on their infrared energy signatures. While these structures would absorb electromagnetic energy (light) from their stars, they would radiate energy in the form of heat (infrared) which could be detected.
A more modern approach involves extra-solar planet research, where Transit Photometry has been used to try and discern the presence of massive, artificial structures around a star. A good example of this is the case of KIC 8462852 (Tabby’s Star), where strange dips in the star’s brightness were seen as a possible indication of a megastructure (though this remains entirely speculative).
Scientists have also recommended using this method to look for massive constellations of artificial satellites around a planet. These rings, known as Clarke Belt’s (named after the late Arthur C. Clarke), would not only indicate the presence of an advanced culture, but one that is capable of communicating with us.
Another technosignature that scientists have considered looking for are signs of industrial activity on a planet. Here too, carbon dioxide would be seen as an indication of an advanced civilization, along with other industrial chemicals (carbon monoxide, ground-level ozone, toxic metals, CFCs, etc.). Radioactive isotopes would also be an indicator, since they could be the result of nuclear testing.
Another possible (and exciting) technosignature would be signs of space travel. For example, rockets being launched from a planet would produce a heat signature that could be detected with instruments of sufficient sensitivity. Interstellar spacecraft could also be detected from the various forms of radiation they emit – from photons to cyclotron radiation – that would be easily discernible from background radiation.
Another possible technosignatures that scientists could be on the lookout for are space-based alien artifacts (or “bottled messages”). These could take the form of spacecraft that contain messages similar to the “Pioneer Plaque” of the Pioneer 10and 11 missions, or the “Golden Record” of the Voyager 1 and 2 missions – both of which are now in interstellar space!
The History and Methods of SETI/METI:
The first known example of SETI is an experiment conducted by Nikola Tesla. In 1896, he proposed using an extreme version of his wireless electrical transmission system to contact a civilization on Mars (which was believed to be possible at the time). In 1899, while conducting experiments at his experimental station in Colorado Springs, he thought he detected a signal from Mars when an odd static signal cut off when Mars set in the sky (which was never confirmed).
Mars continued to be the source of radio surveys for many decades, but without conclusive results. But by the 1950s and 60s, growing interest in space exploration and the advancement of technology allowed for the first SETI projects that targeted other star systems.
For instance, in 1960, Francis Drake conducted the first modern SETI experiment using the radio telescope at the at the National Radio Astronomy Observatory in Green Bank, West Virginia. Known as Project Ozma, this experiment examined Tau Ceti and Epsilon Eridani for radio signals near the 1420 MHz, which corresponds to the frequency of cold hydrogen gas in interstellar space. Unfortunately, Drake and his colleagues found little more than static.
After this project, SETI radio surveys became far more commonplace. In 1971, NASA funded a SETI study that called for the construction of a radio telescope array with 1,500 dishes – known as Project Cyclops. While it was never built, this report formed of the basis of much of the SETI work that followed.

In 1974, the most powerful broadcast ever deliberately sent into space was made from the Arecibo Radio Telescope in Puerto Rico. This was known as the Arecibo Message, and consisted of a simple, pictorial message that was composed by Francis Drake and Carl Sagan. The message was composed of 1,679 binary digits (the product of two prime numbers) and arranged rectangularly in 73 rows by 23 columns (also prime numbers).
The message contains many elements which, in descending order, include the numbers one to ten in white, the atomic numbers of hydrogen, carbon, nitrogen, oxygen, and phosphorus (the building blocks of DNA) in purple, the formulas for the sugars and bases in the nucleotides of DNA (in green), the number of nucleotides in DNA and a graphic of its double helix structure of DNA (white & blue), and a stick-figure depicting the profile of a human.
Also include is the human population of Earth (red, blue/white, & white respectively), a graphic of the Solar System indicating where the signal is coming from (yellow), and a graphic of the Arecibo radio telescope and the dimensions of the antenna dish (purple, white, & blue). The message was aimed at the globular star cluster M13, which is roughly 21,000 light-years from Earth and contains thousands of stars.
There are also the efforts of the Ohio State Radio Observatory, also known as the “Big Ear” Observatory. Originally proposed in 1955 and built in 1957, this flat-plane radio telescope was responsible for one of the most significant detections in the history of SETI research. This occurred on August 15th, 1977, when the observatory received a very strong signal that appeared to be coming from the constellation Sagittarius.
On the following day, volunteer astronomer Jerry Ehman circled the indicated signal on a printout and wrote “WOW!” in the margin. This event, which came to be known as the “WOW! Signal”, is considered by many to be the best candidate for a radio signal detected from a possible extraterrestrial source. Subsequent surveys of the Sagittarius constellation have failed to detect it since.
In 1980, Carl Sagan was joined by Bruce Murray and Louis Friedman from NASA’s Jet Propulsion Laboratory (NASA JPL) to found the U.S. Planetary Society. Created in part as a vehicle for SETI studies, society played a significant role in the development of SETI-related programs and software. This included “Suitcase SETI,” a desktop spectrum analyzer developed by Harvard physicist Paul Horowitz in 1981.
This led to the creation of Sentinel, a project which relied on the 26-meter (85 foot) Harvard/Smithsonian radio telescope at Oak Ridge Observatory and ran from 1983 to 1985. These efforts were followed by the Megachannel Extra-Terrestrial Assay(META) project in 1985 and the Billion-channel Extraterrestrial Assay (BETA) in 1995. In 1999 after a windstorm caused the Harvard/Smithsonian radio telescope to fall over and suffer serious damage.
In 1992, the U.S. government began funding the NASA Microwave Observing Program (MOP), which was planned as a long-term effort to conduct a general survey of the sky and 800 specific nearby stars. This project relied on the radio antennas associated with the NASA Deep Space Network (DSN), as well as the Green Bank Telescope and the 300 m (1000 ft) radio telescope at the Arecibo Observatory in Peurto Rico.

After one year, the U.S. Congress canceled the program and the MOP team was forced to continue without government funding. In 1995, the SETI Institute resurrected the program with private funding and named it Project Phoenix. Between 1995 and 2004, the project conducted observations of 800 stars within a 200 light year radius.
In 2015, citizen astronomers with the Planet Hunters project announced strange behavior coming from KIC 8462852, a star located 1,470 light-years from Earth in the Cygnus constellation. Using data from the Kepler Space Telescope, the team noted a 22% drop in luminosity coming from the star that did not appear to be the result of natural phenomena.
The team published a paper about their findings and the star was nicknamed Tabby’s Star (aka. Boyajian’s Star) after the team leader, Tabetha Boyajian. Between 2015 and 2018, more dips in brightness were recorded and multiple attempts were made to explain the behavior – ranging from transiting comets and a consumed planet to a debris disk, a ring system, and even an alien megastructure.
In 2016, the non-profit organization Breakthrough Initiatives (founded by Russian billionaire Yuri Milner) launched Breakthrough Listen, the largest SETI program to date. Listen combines radio wave observations from the Green Bank Observatory and the Parkes Observatory, and visible light observations from the Automated Planet Finder.

On October 26th, 2016, Breakthrough Listen dedicated eight hours of scope time to Tabby’s Star to “listen” for any signs of radio signals. Follow-ups observations were made in the subsequent months, but no signals were detected.
In the same year, China completed the Five-hundred-meter Aperture Spherical radio Telescope (FAST) – aka. Tianyan, or the “Eye of Heaven”. With a radio antenna dish measuring 500 m (1,600 ft) in diameter, it is the world’s largest filled-aperture radio telescope and will be dedicated to SETI research in the near future.
In 2017, construction was completed on the Canadian Hydrogen Intensity Mapping Experiment (CHIME). This interferometric radio telescope, which is located the Dominion Radio Astrophysical Observatory (DRAO) in British Columbia, will be intrinsic to the study of Fast Radio Bursts (FRBs).
Examples of ongoing radio surveys include the SETI Institute’s Allen Telescope Array, the Arecibo Observatory, the Robert C. Byrd Green Bank Telescope, the Parkes Telescope, and the Very Large Array (VLA), the SETI@home project and Breakthrough Listen.

Examples of ongoing optical and near-infrared light (NIL) surveys include the Near-Infrared Optical SETI (NIROSETI) instrument, the Very Energetic Radiation Imaging Telescope Array System (VERITAS), the Near-Earth Object Wide-field Survey Explorer (NEOWISE), and the Keck/High Resolution Echelle Spectrometer (HIRES).
Similarly, surveys have been conducted of other galaxies using the Wide-field Infrared Survey Explorer (WISE) and Two Micron All-Sky Survey (2MASS). Other ongoing searches are being conducted with the Infrared Astronomical Satellite(IRAS) and the Vanishing & Appearing Sources during a Century of Observations(VASCO).
Current Limitations and the Future of SETI:
So far, all of humanity’s efforts to find evidence of extra-terrestrial life or ETIs have yielded nothing concrete. While there are some examples of cosmic phenomena that continue to defy explanation (the WOW! Signal, FRBs, and perhaps Tabby’s Star), no compelling evidence has been found and the Fermi Paradox still holds.
Part of the reasons for this has to do with the instruments and methods that humanity has been relying on until now. For example, as the NASA Technosignature Workshop report indicated, the current upper limits on radio wave signatures are quite weak. This is due to a combination of the vastness of space and the fact that any artificial radio sources would have to be extremely narrowband (while broadband radio transmissions are a common occurrence in our galaxy).

In addition, our ability to observe distant planets is also limited. While astronomers have confirmed the existence of almost 4000 exoplanets in recent decades, the vast majority were discovered through indirect methods. This has prevented scientists from being able to study exoplanets in greater detail, which has imposed limits on the search for biosignatures and technosignatures.
But perhaps the greatest limitations are conceptual. As noted several times already, astronomers are forced to look for life and technology “as we know it”. While this does allow us to narrow the search considerably, it’s also very limiting. As it stands, humanity knows of only one planet and one set of conditions under which life can exist – Earth, a rocky planet located within the “Goldilocks Zone” of our Sun.
In accordance with the most widely accepted theories, life began on Earth in the form of simple bacteria. While our atmosphere was initially composed of a toxic plume of carbon dioxide and methane, the presence of photosynthetic bacteria eventually converted it to one composed of nitrogen and oxygen gas. Tectonic activity is also thought to have played a significant role in the emergence and evolution of life.
It is little wonder then why the hunt for “potentially-habitable planets” around distant stars is focused on rocky planets that orbit close enough to their stars to support liquid water on their surfaces. Given our current limitations, both technologically and conceptually, scientists have only been able to look for signs associated with life, rather than life itself.
However, much of that is likely to change in the coming years and decades, thanks in no small part to the deployment of next-generation telescopes. These include the James Webb Space Telescope (JWST), which is scheduled to launch by 2021. As the largest space telescope ever deployed and with superior instruments, the JWST will be able to conduct infrared astronomy with far greater sensitivity.
Another space telescope that is currently under development is NASA’s Wide Field Infrared Survey Telescope (WFIRST). As a successor to Hubble, the WFIRST will capture Hubble-quality images covering swaths of sky 100 times larger than its predecessor did. Its advanced suite of instruments will also allow it to conduct near-infrared surveys of the sky in order to answer fundamental questions about the structure and evolution of the Universe and greatly expand our knowledge of planetary systems around other stars.
The PLAnetary Transits and Oscillations of stars (PLATO) mission is another planned space telescope currently under development by the European Space Agency (ESA) and scheduled for deployment in 2026. This mission will search for planetary transits across up to one million stars to discover and characterize rockyextrasolar planets around a variety of stars – including red dwarfs, subgiants, and yellow dwarf stars (like our Sun).
The search for extra-terrestrial civilizations will also benefit from the introduction of next-generation ground telescopes that incorporate adaptive optics instruments (which compensate for atmospheric interference). These include the European Southern Observatory’s Extremely Large Telescope (ELT), which is currently under construction in Chile and is expected to be complete in 2025.
There’s also the Thirty Meter Telescope (TMT), which has been approved for construction on Mauna Kea, Hawaii – and is expected to be finished by the mid-2020s – and the Giant Magellan Telescope (GMT) that is under construction at the Las Campanas Observatory and scheduled for completion in 2025.
These instruments, with their increased sensitivity, adaptive optics and advanced instruments (a coronograph and a suite of spectrometers and imagers) are expected to enable the study of extrasolar planets using Direct Imaging. This will allow scientists to study the atmospheres of “potentially habitable” planets and discern the presence of biosignatures and technosignatures like never before.
Unfortunately, not much can be done about our conceptual framework for finding life. Seeing as how humanity is armed with only one example of a life-bearing planet (Earth) and one technologically-advanced civilization (our own), our efforts are likely to remain restricted to searching for “life as we know it”.
But that’s the good thing about the Fermi Paradox: it only needs to be resolved once! Essentially, all we need to do is find one example of life beyond our Solar System and we will know what other kinds of life to be on the lookout for. We will also know with certainty that humanity is not alone in the Universe!
Source: Interesting Engineering